The Definition of Biophysics: What Exactly is Biophysics?
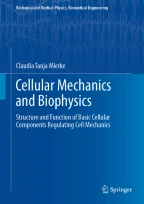
This chapter describes what kind of research is performed in biophysics and what major breakthroughs biophysics has brought. The big problem for the field of biology and life sciences is that we have too much information about all molecules and their signaling pathways. We are simply lost in all the information and cannot decipher the essential parts. Thus, we need to focus on the framework helping us to organize and categorize all those endless numbers of facts. Biologists and life scientists wonder why a physicist is often seen as a reductionist who wants to leave out all the details that distinguish cells from metals. The reason for the reduction is that the whole unified framework must finally be seen in the context of an overall picture. The reduction to the main characteristics stands in contrast to the diversity and abundance of the mechanisms and interaction pathways that provide a fruitful, but also enormous tension. Thus, future research will at the first glance combine these seemingly contradictory directions and, if necessary, switch back and forth between them in order to understand these living beings. There has been a tremendous development of several physical techniques suitable for the measuring of living biological matter, and there is an approach revealing what happens in the nano- and microenvironment of cells. Moreover, a lot of physical ideas and principles are behind the scientific cartoons and schematic drawings present in cell biology and molecular biology books, which may serve as suitable tests to confirm or reject these hypotheses. In general, the biological question of how living organisms can be highly ordered differs from the physical question of whether the flow of energy can evoke increased order. Basically, both scientists, the biologist and physicist, achieve the same thing, but have very different approaches to getting answers and reaching their goals. In detail, this chapter gives a brief overview of the field of biophysics and also mentions subareas. The main objective of this chapter is to develop an understanding of biological processes and mechanisms and to sensitize the reader to the great diversity observed in the study of living materials in biological processes that can be attained in all fields of biology.
This is a preview of subscription content, log in via an institution to check access.
Access this chapter
Subscribe and save
Springer+ Basic
€32.70 /Month
- Get 10 units per month
- Download Article/Chapter or eBook
- 1 Unit = 1 Article or 1 Chapter
- Cancel anytime
Buy Now
Price includes VAT (France)
eBook EUR 192.59 Price includes VAT (France)
Softcover Book EUR 242.64 Price includes VAT (France)
Hardcover Book EUR 242.64 Price includes VAT (France)
Tax calculation will be finalised at checkout
Purchases are for personal use only
References
- R.M. Addoms, Toxicity as evidenced by changes in the protoplasmic structure of root hairs of wheat. Am. J. Bot. 14, 147–165 (1927) ArticleGoogle Scholar
- J. Alcaraz, L. Buscemi, M. Grabulosa, X. Trepat, B. Fabry, R. Farre, D. Navajas, Microrheology of human lung epithelial cells measured by atomic force microscopy. Biophys. J. 84, 2071–2079 (2003) ArticleADSGoogle Scholar
- C.A. Angerer, The effect of electric current on the relative viscosity of sea-urchin egg protoplasm. Bio Bull. 77, 399–406 (1939) ArticleGoogle Scholar
- G. Bao, S. Suresh, Cell and molecular mechanics of biological materials. Nat. Mater. 2, 715–725 (2003) ArticleADSGoogle Scholar
- W.M. Bayliss, The properties of colloidal systems. IV Reversible gelation in living protoplasm. Proc. R. Soc. London B. 91, 196–201 (1920) ArticleADSGoogle Scholar
- J. Bernstein, Untersuchungen zur Thermodynamik der bio-elektrischen Ströme. Arch f. Physiologie 92, 521–562 (1902) ArticleGoogle Scholar
- E.C. Bingham, Fluidity and Plasticity, 1st edn. (McGraw- Hill, New York, 1933) Google Scholar
- G. Binnig, C.F. Quate, C. Gerber, Atomic force microscope. Phys. Rev. Lett. 56, 930–933 (1986) ArticleADSGoogle Scholar
- I.B. Bischofs, U.S. Schwarz, Cell organization in soft media due to active mechanosensing. Proc. Natl. Acad. Sci. U. S. A. 100, 9274–9279 (2003) ArticleADSGoogle Scholar
- I.B. Bischofs, U.S. Schwarz, Effect of Poisson ratio on cellular structure formation. Phys. Rev. Lett. 95, 068102 (2005) ArticleADSGoogle Scholar
- I.B. Bischofs, U.S. Schwarz, collective effects in cellular structure formation mediated by compliant environments: a Monte Carlo study. Acta Biomater. 2, 253–265 (2006) ArticleGoogle Scholar
- D.H. Boal, Mechanics of the Cell, 2nd edn. (Cambridge University Press, Cambridge, UK, 2002) Google Scholar
- M.R. Branco, A. Pombo, Intermingling of chromosome territories in interphase suggests role in translocations and transcription-dependent associations. PLoS Biol. 4, e138 (2006) ArticleGoogle Scholar
- C.P. Brangwynne, G.H. Koenderink, F.C. MacKintosh, D.A. Weitz, Cytoplasmic diffusion: molecular motors mix it up. J Cell Biol. 183, 583–587 (2008) ArticleGoogle Scholar
- D. Bray, Axonal growth in response to experimentally applied mechanical tension. Dev. Biol. 102, 379–389 (1984) ArticleGoogle Scholar
- J.G. Carlson, Protoplasmic viscosity changes in different regions of the grasshopper neuroblast during mitosis. Bio Bull. 90, 109–121 (1946) ArticleGoogle Scholar
- R. Chambers, Microdissection studies on the germ cell. Science 41, 290–293 (1915) ArticleADSGoogle Scholar
- R. Chambers, H.B. Fell, Micro-operations on cells in tissue cultures. Proc. R. Soc. London B 109, 380–403 (1931) ArticleADSGoogle Scholar
- G.T. Charras, M.A. Horton, Single cell mechanotransduction and its modulation analyzed by atomic force microscope indentation. Biophys. J. 82, 2970–2981 (2002) ArticleADSGoogle Scholar
- G.T. Charras, P.P. Lehenkari, M.A. Horton, Atomic force microscopy can be used to mechanically stimulate osteoblasts and evaluate cellular strain distributions. Ultramicroscopy 86, 85–95 (2001) ArticleGoogle Scholar
- G.T. Charras, J.C. Yarrow, M.A. Horton, L. Mahadevan, T.J. Mitchison, Non-equilibration of hydrostatic pressure in blebbing cells. Nature 435, 365–369 (2005) ArticleADSGoogle Scholar
- C. Chaubaroux, F. Perrin-Schmitt, B. Senger, L. Vidal, J.C. Voegel, P. Schaaf, Y. Haikel, F. Boulmedais, P. Lavalle, J. Hemmerlé, Cell alignment driven by mechanically induced collagen fiber alignment in collagen/alginate coatings. Tissue Eng. Part C Methods 21, 881–888 (2015) ArticleGoogle Scholar
- A.E. Cohen, Optogenetics: turning the microscope on its head. Biophys. J. 110, 997–1003 (2016) Google Scholar
- P.F. Cranefield, The organic physics of 1847 and the biophysics of today. J. Hist. Med. Allied Sci. 12, 407–423 (1957) ArticleGoogle Scholar
- J. Dai, M.P. Sheetz, Cell membrane mechanics. Methods Cell. Biol. 55, 157–171 (1998) ArticleGoogle Scholar
- B. Daily, E.L. Elson, G.I. Zahalak, Cell poking. Determination. of the elastic area compressibility modulus of the erythrocyte membrane. Biophys. J.45, 671–82 (1984) Google Scholar
- R. De, A. Zemel, S.A. Safran, Dynamics of cell orientation. Nat. Phys. 3, 655–659 (2007) ArticleGoogle Scholar
- J. Domke, W.J. Parak, M. George, H.E. Gaub, M. Radmacher, Mapping the mechanical pulse of single cardiomyocytes with the atomic force microscope. Eur. J. Biophys. 28, 179–186 (1999) ArticleGoogle Scholar
- T.L. Downing, J. Soto, C. Morez, T. Houssin, A. Fritz, F. Yuan, J. Chu, S. Patel, D.V. Schaffer, S. Li, Biophysical regulation of epigenetic state and cell reprogramming. Nat. Mater. 12, 1154–1162 (2013) ArticleADSGoogle Scholar
- R.J. Dyson, J.E. Green, J.P. Whiteley, H.M. Byrne, An investigation of the influence of extracellular matrix anisotropy and cell-matrix interactions on tissue architecture. J. Math. Biol. 72, 1775–1809 (2016) ArticleMathSciNetMATHGoogle Scholar
- M. Eastwood, V.C. Mudera, D.A. McGrouther, R.A. Brown, Effect of precise mechanical loading on fibroblast populated collagen lattices: morphological changes. Cell Motil. Cytoskeleton. 40, 13–21 (1998) ArticleGoogle Scholar
- A.J. Engler, S. Sen, H.L. Sweeney, D.E. Discher, Matrix elasticity directs stem cell lineage specification. Cell 126, 677–689 (2006) ArticleGoogle Scholar
- E.A. Evans, R.M. Hochmuth, Membrane viscoelasticity. Biophys. J. 16, 1–11 (1976) ArticleGoogle Scholar
- E. Evans, A. Yeung, Apparent viscosity and cortical tension of blood granulocytes determined by micropipet aspiration. Biophys. J. 56, 151–160 (1989) ArticleADSGoogle Scholar
- S. Even-Ram, V. Artym, K.M. Yamada, Matrix control of stem cell fate. Cell 126, 645–647 (2006) ArticleGoogle Scholar
- A.J. Ewart, On the physics and physiology of the protoplasmic streaming in plants. Proc. R. Soc. London 69, 466–470 (1901) Google Scholar
- D.A. Fletcher, R.D. Mullins, Cell mechanics and the cytoskeleton. Nature 463(7280), 485–492 (2010) ArticleADSGoogle Scholar
- A. Forbes, C. Thacher, Changes in the protoplasm of Nereis eggs induced by ß-radiation. Am. J. Physiol. 74, 567–578 (1925) ArticleGoogle Scholar
- L. Formigli, E. Meacci, C. Sassoli, F. Chellini, R. Giannini, F. Quercioli, B. Tiribilli, R. Squecco, P. Bruni, F. Francini, S. Zecchi-Orlandini, Sphingosine 1-phosphate induces cytoskeletal reorganization in C2C12 myoblasts: physiological relevance for stress fibres in the modulation of ion current through stretch-activated channels. J Cell. Sci. 118, 1161–1171 (2005) Google Scholar
- A. Greely, Experiments on the physical structure of the protoplasm of Paramaecium and its relation to the reactions of the organism to thermal, chemical and electrical stimuli. Biol. Bull. 7, 3–32 (1904) Google Scholar
- J. Guck, R. Ananthakrishnan, H. Mahmood, T.J. Moon, C.C. Cunningham, J. Kas, The optical stretcher: a novel laser tool to micromanipulate cells. Biophys. J. 81, 767–784 (2001) ArticleGoogle Scholar
- J. Guck, S. Schinkinger, B. Lincoln, F. Wottawah, S. Ebert, M. Romeyke, D. Lenz, H.M. Erickson, R. Ananthakrishnan, D. Mitchell, J. Kas, S. Ulvick, C. Bilby, Optical deformability as an inherent cell marker for testing malignant transformation and metastatic competence. Biophys. J. 88, 3689–3698 (2005) ArticleGoogle Scholar
- B.J. Haupt, A.E. Pelling, M.A. Horton, Integrated confocal and scanning probe microscopy for biomedical research. Sci. World J. 6, 1609–1618 (2006) Google Scholar
- S.R. Heidemann, P. Lamoureaux, R.E. Buxbaum, Opposing views on tensegrity as a structural framework for understanding cell mechanics. J. Appl. Physiol. 89, 1670–1678 (2000) Google Scholar
- L.V. Heilbrunn, The physical effect of anesthetics upon living protoplasm. Bio Bull. 39, 307–315 (1920) ArticleGoogle Scholar
- L.V. Heilbrunn, An experimental study of cell division. I the physical conditions which determine the appearance of the spindle in sea-urchin eggs. J. Exp. Zool. 30, 211–237 (1920) ArticleGoogle Scholar
- L.V. Heilbrunn, The surface tension theory of membrane elevation. Bio Bull. 46, 277–280 (1924) ArticleGoogle Scholar
- L.V. Heilbrunn, The electrical charges of living cells. Science 1574, 236–237 (1925) ArticleADSGoogle Scholar
- L.V. Heilbrunn, The action of ether on protoplasm. Bio Bull 49, 461–476 (1925) ArticleGoogle Scholar
- L.V. Heilbrunn, The physical structure of the protoplasm of sea-urchin eggs. Am. Nat. 60, 143–156 (1926) ArticleGoogle Scholar
- L.V. Heilbrunn, The viscosity of the protoplasm. Q. Rev. Biol. 2, 230–248 (1927) ArticleGoogle Scholar
- L.V. Heilbrunn, W.L. Wilson, A rational approach to the problem of cancer chemotherapy. Bio Bull. 113, 388–396 (1957) Google Scholar
- L.V. Heilbrunn, A.B. Chaet, A. Dunn, W.L. Wilson, Antimitotic substances from ovaries. Bio Bull. 106, 158–168 (1954) ArticleGoogle Scholar
- L.V. Heilbrunn, W.L. Wilson, Protoplasmic viscosity changes during mitosis in the egg of chaetopterus. Bio Bull. 95, 57–68 (1948) ArticleGoogle Scholar
- L.V. Heilbrunn, W.L. Wilson, T.R. Tosteson, E. Davidson, R.J. Rutman, The antimitotic and carcinostatic action of ovarian extracts. Bio Bull. 113, 129–134 (1957) ArticleGoogle Scholar
- E.H. Herrick, Mechanism of movement of epidermis, especially its melanophores, in wound healing, and behavior of skin grafts in frog tadpoles. Bio Bull.63, 271–286 (1932) Google Scholar
- A.V. Hill, The possible effects of the aggregation of the molecules of hemoglobin on its dissociation curves. J. Physiol. 40, iv–vii (1910) Google Scholar
- A.V. Hill, Why biophysics? Science 124, 1233–1237 (1956) ArticleADSGoogle Scholar
- R.M. Hochmuth, Micropipette aspiration of living cells. J. Biomech. 33, 15–22 (2000) ArticleGoogle Scholar
- R. Horwitz, Cellular biophysics. Biophys. J. 110, 993–996 (2016) ArticleADSGoogle Scholar
- S. Hu, L. Eberhard, J. Chen, J.C. Love, J.P. Butler, J.J. Fredberg, G.M. Whitesides, N. Wang, Mechanical anisotropy of adherent cells probed by a three-dimensional magnetic twisting device. Am. J. Physiol. Cell Physiol. 287, C1184–C1191 (2004) ArticleGoogle Scholar
- H. Huang, R.D. Kamm, R.T. Lee, Cell mechanics and mechanotransduction: pathways, probes, and physiology. Am. J. Physiol. Cell Physiol. 287, C1–C11 (2004) ArticleGoogle Scholar
- D.E. Ingber, Opposing views on tensegrity as a structural framework for understanding cell mechanics. J. Appl. Physiol. 89, 1663–1670 (2000) ArticleGoogle Scholar
- D.E. Ingber, Mechanical control of tissue growth: function follows form. Proc. Natl. Acad. Sci. U. S. A. 102, 11571–11572 (2005) ArticleADSGoogle Scholar
- D.E. Ingber, Mechanical control of tissue morphogenesis during embryological development. Int. J. Dev. Biol. 50, 255–266 (2006) ArticleGoogle Scholar
- M.H. Jacobs, The effect of carbon dioxide on the consistency of protoplasm. Bio Bull. 42, 14–30 (1922) ArticleGoogle Scholar
- C. Jurado, J.R. Haserick, J. Lee, Slipping or gripping? Fluorescent speckle microscopy in fish keratocytes reveals two different mechanisms for generating a retrograde flow of actin. Mol. Biol. Cell 16, 507–518 (2005) ArticleGoogle Scholar
- K.E. Kasza, A.C. Rowat, J. Liu, T.E. Angelini, C.P. Brangwynne, G.H. Koenderink, D.A. Weitz, The cell as a material. Curr. Opin. Cell Biol. 19, 101–107 (2007) ArticleGoogle Scholar
- G.L. Kite, Studies on the physical properties of protoplasm. Am. J. Physiol. 32, 146–164 (1913) ArticleGoogle Scholar
- R.J. Klebe, H. Caldwell, S. Milam, Cells transmit spatial information by orienting collagen fibers. Matrix 9, 451–458 (1989) ArticleGoogle Scholar
- W.A. Lam, M.J. Rosenbluth, D.A. Fletcher, Chemotherapy exposure increases leukemia cell stiffness. Blood 109, 3505–3508 (2007) ArticleGoogle Scholar
- J. Lammerding, K.N. Dahl, D.E. Discher, R.D. Kamm, Nuclear mechanics and methods. Methods Cell Biol. 83, 269–294 (2007) ArticleGoogle Scholar
- J. Lammerding, L.G. Fong, J.Y. Ji, K. Reue, C.L. Stewart, S.G. Young, R.T. Lee, Lamins A and C but not lamin B1 regulate nuclear mechanics. J. Biol. Chem.281, 25768–25780 Google Scholar
- J. Lammerding, P.C. Schulze, T. Takahashi, S. Kozlov, T. Sullivan, R.D. Kamm, C.L. Stewart, R.T. Lee, Lamin A/C deficiency causes defective nuclear mechanics and mechanotransduction. J. Clin. Invest. 113, 370–378 (2004) ArticleGoogle Scholar
- E.J. Lee, J.W. Holmes, K.D. Costa, Remodeling of engineered tissue anisotropy in response to altered loading conditions. Ann. Biomed. Eng. 36, 1322–1334 (2008) ArticleGoogle Scholar
- T.P. Lele, J.E. Sero, B.D. Matthews, S. Kumar, S. Xia, M. Montoya- Zavala, T. Polte, D. Overby, N. Wang, D.E. Ingber, Tools to study cell mechanics and mechanotransduction. Methods Cell. Biol. 83, 443–472 (2007) Google Scholar
- W.W. Lepeschkin, The influence of narcotics, mechanical agents, and light upon the permeability of protoplasm. Am. J. Bot.19, 568–580 (1932) Google Scholar
- S. Li, J.L. Guan, S. Chien, Biochemistry and biomechanics of cell motility. Annu. Rev. Biomed. Eng. 7, 105–150 (2005) ArticleGoogle Scholar
- C. Liu, S. Baek, J. Kim, E. Vasko, R. Pyne, C. Chan, Effect of static pre-stretch induced surface anisotropy on orientation of mesenchymal stem cells. Cell Mol. Bioeng. 7, 106–121 (2014) ArticleGoogle Scholar
- J.M. Maloney, D. Nikova, F. Lautenschläger, E. Clarke, R. Langer, J. Guck, K.J. VanVliet, Mesenchymal stem cell mechanics from the attached to the suspended state. Biophys. J. 99, 2479–2487 (2010) ArticleADSGoogle Scholar
- T.G. Mason, K. Ganesan, J.H. vanZanten, D. Wirtz, S.C. Kuo, Particle tracking microrheology of complex fluids. Phys. Rev. Lett. 79, 3282–3285 (1997) ArticleADSGoogle Scholar
- G. Massiera, K.M. Van Citters, P.L. Biancaniello, J. Crocker, Mechanics of single cells: rheology, time dependence and fluctuations. Biophys. J.93, 3703–3713 (2007) Google Scholar
- H. Matsuda, G.G. Putzel, V. Backman, I. Szleifer, Macromolecular crowding as a regulator of gene transcription. Biophys. J. 106, 1801–1810 (2014) ArticleADSGoogle Scholar
- W.B. McConnaughey, N.O. Petersen, Cell poker: an apparatus for stress–strain measurements on living cells. Rev. Sci. Instrum.51, 575–580 (1980) Google Scholar
- A.D. McCulloch, Systems biophysics: multiscale biophysical modeling of organ systems. Biophys. J. 110(5), 1023–1027 (2016) ArticleADSGoogle Scholar
- C.T. Mierke, Cancer cells regulate biomechanical properties of human microvascular endothelial cells. J. Biol. Chem. 286, 40025–40037 (2011) ArticleGoogle Scholar
- C.T. Mierke, Phagocytized beads reduce the α5β1 integrin facilitated invasiveness of cancer cells by regulating cellular stiffness. Cell Biochem. Biophys. 66, 599–622 (2013) Google Scholar
- C.T. Mierke, The matrix environmental and cell mechanical properties regulate cell migration and contribute to the invasive phenotype of cancer cells. Rep. Prog. Phys. 82(6), 064602 (2019) ArticleADSGoogle Scholar
- C.T. Mierke, The role of the optical stretcher is crucial in the investigation of cell mechanics regulating cell adhesion and motility. Front. Cell Dev. Biol. 7, 184 (2019) ArticleGoogle Scholar
- C.T. Mierke, N. Bretz, P. Altevogt, Contractile forces contribute to increased GPI-anchored receptor CD24 facilitated cancer cell invasion. J. Biol. Chem. 286, 34858–34871 Google Scholar
- C.T. Mierke, T. Fischer, S. Puder, T. Kunschmann, B. Soetje, W.H. Ziegler, Focal adhesion kinase activity is required for actomyosin contractility-based invasion of cells into dense 3D matrices. Sci. Rep.7, 42780 (2017) Google Scholar
- C.T. Mierke, B. Frey, M. Fellner, M. Herrmann, B. Fabry, Integrin α5β1 facilitates cancer cell invasion through enhanced contractile forces. J. Cell Sci. 124, 369–83 (2011) Google Scholar
- C.T. Mierke, P. Kollmannsberger, D. Paranhos-Zitterbart, G. Diez, T.M. Koch, S. Marg, W.H. Ziegler, W.H. Goldmann, B. Fabry, Vinculin facilitates cell invasion into 3D collagen matrices. J. Biol. Chem. 285, 13121–13130 (2010) Google Scholar
- C.T. Mierke, P. Kollmannsberger, D.P. Zitterbart, J. Smith, B. Fabry, W.H. Goldmann, Mechano-coupling and regulation of contractility by the vinculin tail domain. Biophys. J. 94(2), 661–670 (2008) ArticleGoogle Scholar
- C.T. Mierke, D.P. Zitterbart, P. Kollmannsberger, C. Raupach, U. Schlotzer-Schrehardt, T.W. Goecke, J. Behrens, B. Fabry, Breakdown of the endothelial barrier function in tumor cell transmigration. Biophys. J. 94, 2832–2846 (2008) ArticleGoogle Scholar
- E. Moeendarbary, A.R. Harris, Cell mechanics: principles, practices, and prospects. WIREs Syst. Biol. Med. 6, 371–388 (2014) Google Scholar
- M.R.K. Mofrad, R.D. Kamm, Cytoskeletal Mechanics: Models and Measurements, 1st edn. (Cambridge University Press, Cambridge, 2006) Google Scholar
- D.A. Moulding, E. Moeendarbary, L. Valon, J. Record, G.T. Charras, A.J. Thrasher, Excess F-actin mechanically impedes mitosis leading to cytokinesis failure in X-linked neutropenia by exceeding Aurora B kinase error correction capacity. Blood 120, 3803–3811 (2012) ArticleGoogle Scholar
- V.C. Mudera, R. Pleass, M. Eastwood, R. Tarnuzzer, G. Schultz, P. Khaw, D.A. McGrouther, R.A. Brown, Molecular responses of human dermal fibroblasts to dual cues: contact guidance and mechanical load. Cell Motil. Cytoskeleton 45, 1–9 (2000) ArticleGoogle Scholar
- M. Nicodemi, A. Pombo, Models of chromosome structure. Curr. Opin. Cell Biol. 28, 90–95 (2014) ArticleGoogle Scholar
- H.T. Northern, Alterations in the structural viscosity of protoplasm by colchicine and their relationship to C-mitosis and C-tumor formation. Am. J. Bot. 37, 705–711 (1950) ArticleGoogle Scholar
- I. Obataya, C. Nakamura, S. Han, N. Nakamura, J. Miyake, Nanoscale operation of a living cell using an atomic force microscope with a nanoneedle. Nano Lett. 5, 27–30 (2005) ArticleADSGoogle Scholar
- T. Ochalek, F.J. Nordt, K. Tullberg, M.M. Burger, Correlation between cell deformability and metastatic potential in B16-F1 melanoma cell variants. Cancer Res.48, 5124–5128 (1988) Google Scholar
- C. Packard, The biological effects of short radiations. Q. Rev. Biol. 6, 253–280 (1931) ArticleGoogle Scholar
- J. Paget, Croonian lecture: on the cause of the rhythmic motion of the heart. Proc. R. Soc. London 8, 473–488 (1857) ArticleGoogle Scholar
- Y. Pang, X. Wang, D. Lee, H.P. Greisler, Dynamic quantitative visualization of single cell alignment and migration and matrix remodeling in 3-D collagen hydrogels under mechanical force. Biomaterials 32, 3776–3783 (2011) Google Scholar
- Y. Park, C.A. Best, K. Badizadegan, R.R. Dasari, M.S. Feld, T. Kuriabova, M.L. Henle, A.J. Levine, G. Popescu, Measurement of red blood cell mechanics during morphological changes. Proc. Natl. Acad. Sci. U. S. A. 107, 6731–6736 (2010) ArticleADSGoogle Scholar
- M.J. Paszek, N. Zahir, K.R. Johnson, J.N. Lakins, G.I. Rozenberg, A. Gefen, C.A. Reinhart-King, S.S. Margulies, M. Dembo, D. Boettiger, D.A. Hammer, V.M. Weaver, Tensional homeostasis and the malignant phenotype. Cancer Cell 8, 241–254 (2005) ArticleGoogle Scholar
- K. Pearson, The Grammar of Science, 2nd edn (Adam and Charles Black, London, 1900) Google Scholar
- R.J. Pelham Jr, Y. Wang, High resolution detection of mechanical forces exerted by locomoting fibroblasts on the substrate. Mol. Biol. Cell. 10, 935–945 (1999) ArticleGoogle Scholar
- A.E. Pelling, D.W. Dawson, D.M. Carreon, J.J. Christiansen, R.R. Shen, M.A. Teitell, J.K. Gimzewski, Distinct contributions of microtubule subtypes to cell membrane shape and stability. Nanomedicine 3, 43–52 (2007) ArticleGoogle Scholar
- A.E. Pelling, F.S. Veraitch, C. Pui-Kei Chu, B.M. Nicholls, A.L. Hemsley, C. Mason, M.A. Horton, Mapping correlated membrane pulsations and fluctuations in human cells. J. Mol. Recognit. 20, 467–475 (2007) ArticleGoogle Scholar
- N.O. Petersen, W.B. McConnaughey, E.L. Elson, Dependence of locally measured cellular deformability on position on the cell, temperature, and cytochalasin B. Proc. Natl. Acad. Sci. U. S. A. 79, 5327–5331 Google Scholar
- M. Prass, K. Jacobson, A. Mogilner, M. Radmacher, Direct measurement of the lamellipodial protrusive force in a migrating cell. J. Cell. Biol. 174, 767–772 (2006) ArticleGoogle Scholar
- M. Puig-De-Morales, M. Grabulosa, J. Alcaraz, J. Mullol, G.N. Maksym, J.J. Fredberg, D. Navajas, Measurement of cell microrheology by magnetic twisting cytometry with frequency domain demodulation. J. Appl. Physiol. 91, 1152–1159 (2001) ArticleGoogle Scholar
- M. Radmacher, Measuring the elastic properties of living cells by the atomic force microscope. Methods Cell. Biol. 68, 67–90 (2002) ArticleGoogle Scholar
- M. Radmacher, Studying the mechanics of cellular processes by atomic force microscopy. Methods Cell. Biol. 83, 347–372 (2007) ArticleGoogle Scholar
- J.W. Reinhardt, K.J. Gooch, Agent-based modeling traction force mediated compaction of cell-populated collagen gels using physically realistic fibril mechanics. J. Biomech. Eng. 136, 021024 (2014) ArticleGoogle Scholar
- J.W. Reinhardt, D.A. Krakauer, K.J. Gooch, Complex matrix remodeling and durotaxis can emerge from simple rules for cell-matrix interaction in agent-based models. J. Biomech. Eng. 135, 71003 (2013) ArticleGoogle Scholar
- E.G. Rens, R.M.H. Merks, Cell contractility facilitates alignment of cells and tissues to static uniaxial stretch. Biophys. J. 112, 755–766 (2016) Google Scholar
- C. Rotsch, M. Radmacher, Drug-induced changes of cytoskeletal structure and mechanics in fibroblasts: an atomic force microscopy study. Biophys. J. 78, 520–535 (2000) ArticleADSGoogle Scholar
- C. Rotsch, F. Braet, E. Wisse, M. Radmacher, AFM imaging and elasticity measurements on living rat liver macrophages. Cell Biol. Int. 21, 685–696 (1997) ArticleGoogle Scholar
- C. Rotsch, K. Jacobson, M. Radmacher, Dimensional and mechanical dynamics of active and stable edges in motile fibroblasts investigated by using atomic force microscopy. Proc. Natl. Acad. Sci. U. S. A. 96, 921–926 (1999) ArticleADSGoogle Scholar
- A.C. Rowat, J. Lammerding, J.H. Ipsen, Mechanical properties of the cell nucleus and the effect of emerin deficiency. Biophys. J. 91, 4649–4664 (2006) ArticleADSGoogle Scholar
- G. Sagvolden, I. Giaever, E.O. Pettersen, J. Feder, Cell adhesion force microscopy. Proc. Natl. Acad. Sci. U. S. A. 96, 471–476 (1999) ArticleADSGoogle Scholar
- G.W. Scarth, Colloidal changes associated with protoplasmic contraction. Q. J. Exp. Physiol. 14, 99–113 (1924) ArticleGoogle Scholar
- W. Seifriz, Observations on the structure of protoplasm by aid of microdissection. Biol. Bull. 34, 307–324 (1918) ArticleGoogle Scholar
- W. Seifriz, An elastic value of protoplasm, with further observations on the viscosity of protoplasm. J. Exp. Biol. 2, 1–11 (1924) Google Scholar
- W. Seifriz, The structure of protoplasm, Science 1902, 648–649 (1931) Google Scholar
- W. Seifriz, M. Uraguchi, The toxic effects of heavy metals on protoplasm. Am. J. Bot. 28, 191–197 (1941) ArticleGoogle Scholar
- S.G. Shroff, D.R. Saner, R. Lal, Dynamic micromechanical properties of cultured rat atrial myocytes measured by atomic force microscopy. Am. J. Physiol. 269, C286–C292 (1995) ArticleGoogle Scholar
- T. Sikosek, H.S. Chan, Biophysics of protein evolution and evolutionary protein biophysics. J. R. Soc. Interface 11, 20140419 (2014) ArticleGoogle Scholar
- A.E. Smith, Z. Zhang, C.R. Thomas, K.E. Moxham, A.P. Middelberg, The mechanical properties of fs. Proc. Natl. Acad. Sci. U. S. A. 97, 9871–9874 (2000) ArticleADSGoogle Scholar
- B.A. Smith, H. Roy, P. De Koninck, P. Grutter, Y. De Koninck, Dendritic spine viscoelasticity and soft-glassy nature: balancing dynamic remodeling with structural stability. Biophys. J. 92, 1419–1430 (2007) ArticleADSGoogle Scholar
- B.A. Smith, B. Tolloczko, J.G. Martin, P. Grutter, Probing the viscoelastic behavior of cultured airway smooth muscle cells with atomic force microscopy: stiffening induced by contractile agonist. Biophys. J. 88, 2994–3007 (2005) ArticleGoogle Scholar
- D. Stamenovic, N. Rosenblatt, M. Montoya-Zavala, B.D. Matthews, S. Hu, B. Suki, N. Wang, D.E. Ingber, Rheological behavior of living cells is timescale-dependent. Biophys. J. 93, L39–L41 (2007) ArticleGoogle Scholar
- A. Stuart, Three lectures on muscular motion. Philos. Trans. (1638–1775) 40, i–iiv (1738) Google Scholar
- Y. Sun, C.S. Chen, J. Fu, Forcing stem cells to behave: a biophysical perspective of the cellular microenvironment. Annu. Rev. Biophys. 41, 519–542 (2012) ArticleGoogle Scholar
- Y. Sun, L.G. Villa-Diaz, R.H. Lam, W. Chen, P.H. Krebsbach, J. Fu, Mechanics regulates fate decisions of human embryonic stem cells. PLoS ONE 7, e37178 (2012) ArticleADSGoogle Scholar
- S. Suresh, Biomechanics and biophysics of cancer cells. Acta Biomater. 3, 413–438 (2007) ArticleMathSciNetGoogle Scholar
- K. Svoboda, S.M. Block, Biological applications of optical forces. Annu. Rev. Biophys. Biomol. Struct. 23, 247–285 (1994) ArticleGoogle Scholar
- K. Svoboda, C.F. Schmidt, D. Branton, S.M. Block, Conformation and elasticity of the isolated red blood cell membrane skeleton. Biophys. J. 63, 784–793 (1992) ArticleADSGoogle Scholar
- K. Takakuda, Miyairi, Tensile behaviour of fibroblasts cultured in collagen gel. Biomaterials 17, 1393–1397 (1996) Google Scholar
- D.A.W. Thompson, On Growth and Form, 1st edn. (Cambridge University Press, Cambridge, England, 1917) Google Scholar
- O. Thoumine, A. Ott, O. Cardoso, J.J. Meister, Microplates: a new tool for manipulation and mechanical perturbation of individual cells. J. Biochem. Biophys. Methods39, 47–62 (1999) Google Scholar
- A. Tondon, R. Kaunas, The direction of stretch-induced cell and stress fiber orientation depends on collagen matrix stress. PLoS ONE 9, e89592 (2014) ArticleADSGoogle Scholar
- T. Tørring, N.V. Voigt, J. Nangreave, H. Yan, K.V. Gothelf, DNA origami: a quantum leap for self-assembly of complex structures. Chem. Soc. Rev. 40(12), 5636–5646 (2011) ArticleGoogle Scholar
- O. Treitel, Elasticity of plant tissues. Trans. Kans. Acad. Sci. 47, 219–239 (1944) ArticleGoogle Scholar
- X. Trepat, L. Deng, S.S. An, D. Navajas, D.J. Tschumperlin, W.T. Gerthoffer, J.P. Butler, J.J. Fredberg, Universal physical responses to stretch in the living cell. Nature 447, 592–595 (2007) ArticleADSGoogle Scholar
- D. Vader, A. Kabla, D. Weitz, L. Mahadevan, Strain-induced alignment in collagen gels. PLoS ONE 4, 6e5902 (2009) Google Scholar
- P.A. Valberg, D.F. Albertini, Cytoplasmic motions, rheology, and structure probed by a novel magnetic particle method. J. Cell Biol.101, 130–140 (1985) Google Scholar
- M.T. Valentine, Z.E. Perlman, M.L. Gardel, J.H. Shin, P. Matsudaira, T.J. Mitchison, D.A. Weitz, Colloid surface chemistry critically affects multiple particle tracking measurements of biomaterials. Biophys. J. 86, 4004–4014 (2004) ArticleGoogle Scholar
- N. Wang, D.E. Ingber, Control of cytoskeletal mechanics by extracellular matrix, cell shape, and mechanical tension. Biophys. J.66, 2181–2189 (1994) Google Scholar
- Y. Wang, D.E. Discher, Cell Mechanics, 1st edn. (Elsevier Academic, Amsterdam, 2007) Google Scholar
- R. Waugh, E.A. Evans, Thermoelasticity of red blood cell membrane. Biophys. J. 26, 115–131 (1979) ArticleADSGoogle Scholar
- S.C. Weber, A.J. Spakowitz, J.A. Theriot, Nonthermal ATP-dependent fluctuations contribute to the in vivo motion of chromosomal loci. Proc. Natl. Acad. Sci. U. S. A. 109, 7338–7343 (2012) ArticleADSGoogle Scholar
- D. Weihs, T.G. Mason, M.A. Teitell, Bio-microrheology: a frontier in microrheology. Biophys. J.91, 4296–4305 (2006) Google Scholar
- F. Zhang, Y. Wen, X. Guo, CRISPR/Cas9 for genome editing: progress, implications and challenges. Hum. Mol. Genet.23(R1), R40–R46 (2014) Google Scholar
- P.C. Zhang, A.M. Keleshian, F. Sachs, Voltage-induced membrane movement. Nature 413, 428–432 (2001) ArticleADSGoogle Scholar
Author information
Authors and Affiliations
- Faculty of Physics and Earth Sciences, Peter Debye Institute for Soft Matter Physics, Biological Physics, University of Leipzig, Leipzig, Germany Claudia Tanja Mierke
- Claudia Tanja Mierke